Protein Makeover with Custom Amino Acids
With a plug-and-play strategy, researchers engineer proteins with new functions.
Proteins are built by mixing and matching amino acids, but researchers want to create new functions by adding noncanonical amino acids (ncAAs). However, this process often requires complex whole genome editing. This inspired Ahmed Badran, a chemical and synthetic biologist at the Scripps Research Institute, to develop an easier method for adding ncAAs to proteins.
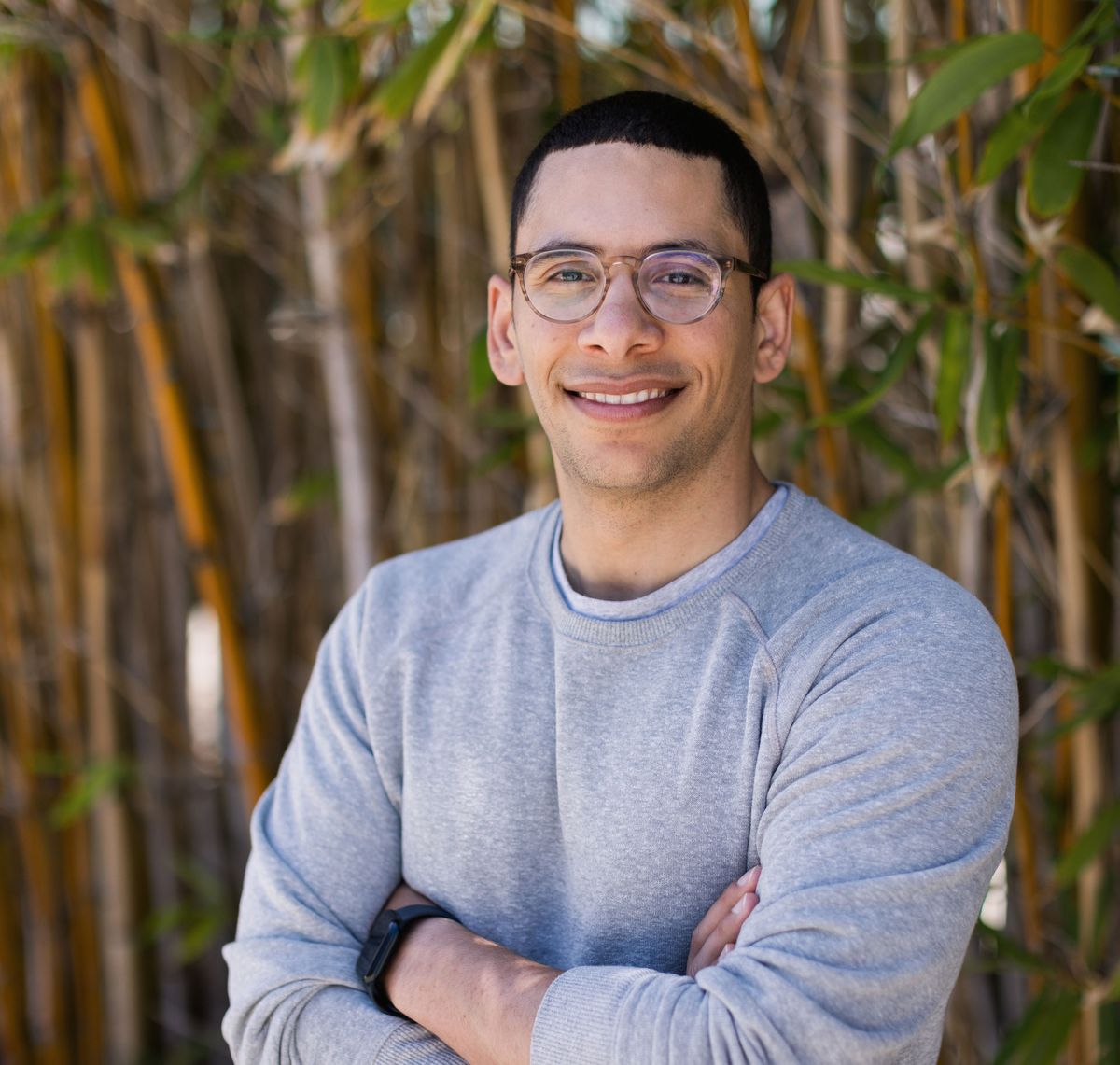
“The assignment of the genetic code has some inherent malleability, which one can change to assign existing codons to new amino acids,” said Badran. His team leveraged this by using a plug-and-play strategy with quadruplet—not the usual triplet—codon translation to insert ncAAs at specific sites without altering the whole genome.
The findings, published in Nature Biotechnology, demonstrated that using sets of four RNA nucleotides could produce more than 100 new cyclic peptides.1 This approach capitalizes on the programmability of proteins to reengineer existing proteins or create entirely new ones.
Badran’s team investigated how codon location and usage affect quadruplet decoding with a modified superfolder green fluorescent protein (sfGFP), replacing a tyrosine codon at position 151 with a quadruplet codon. Screening for sfGFP expression suggested that codons at the downstream plus one position influenced quadruplet decoding and ncAA incorporation efficiency, serving as a location guide for plugging in engineered tRNAs.
During tRNA development, the researchers identified 12 highly active tRNA synthetase/tRNA pairs in Escherichia coli that can add canonical and noncanonical amino acids. Then, Badran’s team used iterative rounds of genetic mutations and screening to enhance the quadruplet-decoding tRNA’s ncAA incorporation ability. They tested five optimized pairs in E.coli, playing with individual or combined pairs, and produced more than 100 new cyclic peptides, each containing up to three ncAAs.
“[The study] is definitely novel,” said Ya-Ming Hou, a biochemist from Thomas Jefferson University, who was not involved in the study, noting the method’s potential to improve protein stability for industrial or medical purposes. “Ultimately, we want to make proteins and enzymes that are diversified with a broad array of noncanonical amino acids,” said Badran.
- Costello A, et al. Nat Biotechnol. 2024.